Scientists use computational modeling to guide a difficult chemical synthesis
Unlocking tough molecules! Study shows how computer modeling guides complex chemical synthesis
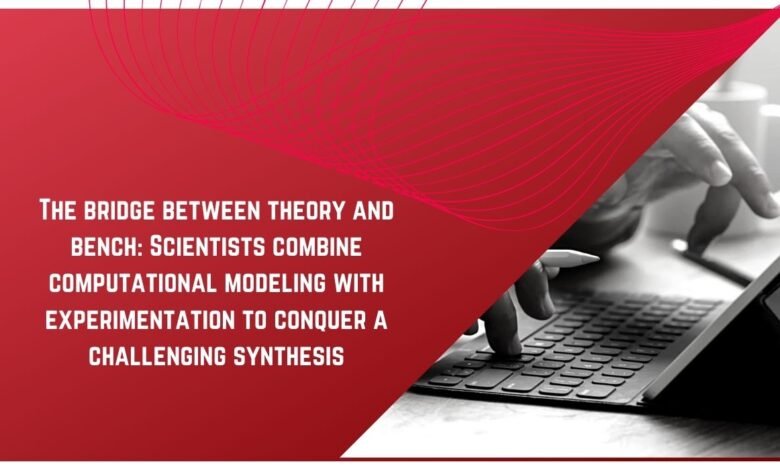
Computational modeling, a pivotal tool in modern chemistry, leverages computer algorithms and simulations to delve into the complexities of chemical reactions. This sophisticated approach enables scientists to gain a deeper understanding of molecular behavior, predict outcomes of chemical processes, and design novel compounds with precision. By utilizing computational modeling, researchers can simulate various scenarios that would be challenging or impossible to observe directly in a laboratory setting.
At its core, computational modeling involves the use of mathematical and physical principles to create detailed models that represent the interactions between atoms and molecules. These models can predict the structure, dynamics, and reactivity of chemical systems, providing invaluable insights that guide experimental efforts. One of the primary advantages of computational modeling is its ability to analyze large datasets and generate predictive models that can forecast chemical behavior under different conditions.
In practical terms, scientists employ a range of computational techniques, such as quantum mechanics, molecular dynamics, and statistical thermodynamics, to simulate chemical reactions. Quantum mechanics, for instance, allows for the calculation of electronic structures and properties of molecules, while molecular dynamics provides a dynamic view of molecular motion over time. These simulations help in visualizing how molecules interact, bond, and transform during chemical reactions.
The significance of computational modeling in chemistry cannot be overstated. It accelerates the discovery of new materials and pharmaceuticals by enabling virtual experimentation, thus reducing the need for extensive trial-and-error laboratory work. Moreover, it aids in the optimization of reaction conditions and the identification of potential reaction pathways, making the synthesis of complex molecules more efficient and targeted.
Computational modeling stands as a cornerstone of contemporary chemical research, offering a powerful means to explore and predict the intricacies of chemical phenomena. Through the integration of computational tools into the scientific process, chemists can achieve a higher level of precision and innovation in their work, ultimately advancing the frontiers of chemical science.
The Challenge of Difficult Chemical Synthesis
Chemical synthesis, the process of creating complex chemical compounds from simpler substances, is a cornerstone of modern science. However, certain chemical reactions are notoriously difficult to achieve, posing significant challenges for researchers. These complex chemical syntheses often involve intricate reaction pathways, sensitive reagents, and precise conditions that must be meticulously controlled.
The challenges in difficult chemical synthesis are multifaceted. One primary issue is the inherent instability of certain intermediates, which can decompose or react undesirably before reaching the desired product. Additionally, some reactions require extreme conditions, such as high temperatures or pressures, which can be both dangerous and resource-intensive. The specificity of reactions is also a critical factor; achieving the correct selectivity to produce the desired compound without generating unwanted by-products is often a daunting task.
Traditional methods of chemical synthesis rely heavily on trial and error, which can be time-consuming and costly. Chemists must painstakingly test various conditions and reagents to identify the optimal pathway for synthesis. This process not only consumes valuable resources but also extends the timeline for achieving successful synthesis, which can delay advancements in fields such as pharmaceuticals, materials science, and agrochemicals.
Given these challenges, there is a pressing need for innovative approaches to streamline and optimize difficult chemical synthesis. Computational modeling has emerged as a powerful tool in this regard, offering the potential to predict and guide chemical reactions with unprecedented accuracy. By leveraging advanced algorithms and high-performance computing, scientists can simulate reaction pathways and identify the most efficient routes to synthesize complex molecules. This not only reduces the need for extensive experimental testing but also enhances the overall efficiency and sustainability of chemical research.
In the face of these complexities, the integration of computational modeling into chemical synthesis represents a significant leap forward, promising to overcome the traditional barriers and accelerate the discovery of new and valuable compounds.
How Computational Modeling Works
Computational modeling is a powerful tool that scientists use to simulate and analyze complex chemical processes. This method allows researchers to predict the behaviors of molecules and reactions without the need for extensive laboratory experiments. Here’s a step-by-step breakdown of how computational modeling works:
1. Creating Models:
- Scientists start by developing a mathematical model that represents the chemical system they want to study. This involves defining the molecular structures, reaction pathways, and relevant physical properties.
- These models are based on fundamental principles of chemistry and physics, such as quantum mechanics and thermodynamics.
2. Running Simulations:
- Once the model is established, simulations are run using specialized software. Popular tools in this field include Gaussian, VASP (Vienna Ab initio Simulation Package), and GROMACS (GROningen MAchine for Chemical Simulations).
- During simulations, the software uses numerical methods to solve the equations governing the chemical processes. This can involve calculating energy levels, reaction rates, and molecular dynamics.
3. Analyzing Results:
- After simulations are completed, scientists analyze the results to gain insights into the chemical behaviors and properties. This analysis helps in understanding reaction mechanisms, predicting product yields, and optimizing synthesis conditions.
- Visualization tools, such as molecular graphics software, are often used to interpret the results graphically, providing a clearer understanding of the molecular interactions.
Computational modeling is not only a theoretical exercise but also a practical approach to guide difficult chemical synthesis. By predicting outcomes and optimizing conditions, it significantly reduces the time and resources required for experimental trials.
Overall, computational modeling serves as a bridge between theoretical chemistry and practical application, enabling scientists to design and execute complex chemical syntheses with greater efficiency and precision.
Benefits of Using Computational Modeling
Computational modeling has become an indispensable tool in the realm of chemical synthesis, offering a plethora of benefits that enhance the efficiency and effectiveness of the process. One primary advantage is its cost-effectiveness. Traditional chemical experiments often require expensive materials and extensive labor, whereas computational models can simulate these experiments at a fraction of the cost. This not only conserves resources but also allows for a broader exploration of potential outcomes without the financial burden.
Time-saving is another critical benefit. Chemical synthesis can be a time-consuming endeavor, often involving numerous trial-and-error experiments. Computational modeling accelerates this process by predicting possible outcomes and narrowing down the most viable pathways. This expedited approach enables scientists to focus their efforts on the most promising experiments, thereby significantly reducing the time required to achieve desired results.
The accuracy of computational modeling further bolsters its value. By utilizing sophisticated algorithms and vast datasets, these models can provide highly precise predictions of molecular behavior and reaction mechanisms. This level of accuracy is instrumental in guiding scientists through complex syntheses, ensuring that each step is meticulously planned and executed. For instance, in the synthesis of complex organic molecules, computational models have been employed to predict the stereochemistry of reactions, leading to more efficient and accurate outcomes.
Moreover, the predictive power of computational modeling cannot be overstated. By simulating various reaction conditions and pathways, these models can forecast the success or failure of a given synthesis, enabling scientists to make informed decisions before committing to lab work. A notable example is the development of new pharmaceuticals, where computational models have successfully predicted the activity and stability of potential drug candidates, thereby streamlining the drug discovery process.
Computational modeling serves as a pivotal tool in chemical synthesis, offering cost savings, time efficiency, unparalleled accuracy, and robust predictive capabilities. These benefits not only enhance the synthesis process but also pave the way for groundbreaking advancements in chemical research and development.
Case Studies: Successful Applications
Computational modeling has been a game-changer in the realm of chemical synthesis, significantly enhancing the ability to predict and optimize complex reactions. Here, we examine specific case studies where computational modeling played a crucial role in overcoming challenging chemical syntheses.
Case Study 1: Synthesis of Complex Natural Products
Natural products often present intricate structures that are difficult to synthesize. One notable example involved the synthesis of a complex marine alkaloid. Traditional methods had failed due to the molecule’s highly stereocentric nature. Researchers turned to computational modeling to:
- Analyze potential reaction pathways
- Predict the most stable intermediates
- Identify the optimal conditions for selectivity
The modeling suggested an alternative pathway which was experimentally validated, leading to a successful synthesis with high yield and selectivity.
Case Study 2: Catalysis Optimization
In another instance, the challenge was to optimize a catalytic process for the industrial synthesis of a pharmaceutical compound. The traditional trial-and-error approach was time-consuming and costly. Computational models were employed to:
- Screen potential catalysts
- Simulate reaction kinetics
- Predict product distribution
The computational approach identified a novel catalyst that significantly increased reaction efficiency, reducing both time and cost.
Case Study 3: Asymmetric Synthesis
Asymmetric synthesis often requires precise control over the generation of chiral centers. A particularly challenging synthesis involved the creation of a chiral drug molecule. Computational modeling was used to:
- Predict enantioselective outcomes
- Analyze transition states
- Optimize reaction conditions for enantioselectivity
The predictions enabled the chemists to achieve high enantiomeric excess, demonstrating the power of computational modeling in guiding asymmetric synthesis.
These case studies underscore the transformative impact of computational modeling in guiding complex chemical syntheses. By predicting reaction pathways, optimizing catalytic processes, and enhancing enantioselectivity, computational models have become indispensable tools for modern chemists.
Limitations and Challenges
While computational modeling has revolutionized chemical synthesis, it is not without its limitations and challenges. One of the primary hurdles is the need for high computational power. Complex chemical reactions often require sophisticated algorithms and extensive computational resources. This demand can make the process time-consuming and cost-prohibitive, particularly for smaller research institutions. Scientists must often rely on supercomputers or cloud-based solutions, which may not always be accessible or affordable for every research team.
Another significant challenge is the potential inaccuracies in computational models. Despite advances in software and algorithms, these models can sometimes produce results that deviate from real-world outcomes. Factors such as incomplete data, oversimplified assumptions, and the inherent complexity of chemical reactions can contribute to these discrepancies. Consequently, models must be rigorously validated against experimental data to ensure their reliability. This validation process can be labor-intensive and requires a close collaboration between computational chemists and experimental scientists.
The requirement for expert knowledge also poses a barrier to the widespread adoption of computational modeling in chemical synthesis. Developing accurate models and interpreting their results necessitates a deep understanding of both chemistry and computational techniques. This expertise is often concentrated in specialized research groups, limiting broader application. To mitigate this, educational initiatives and interdisciplinary collaborations are essential. Training programs that equip chemists with computational skills and vice versa can help bridge this knowledge gap.
Despite these challenges, scientists are actively working to overcome them. Advances in computational power, such as the development of more efficient algorithms and the use of quantum computing, are helping to address the demand for high resources. Efforts to improve the accuracy of models are ongoing, with researchers continually refining algorithms and incorporating more comprehensive data sets. Additionally, initiatives to democratize access to computational tools and foster interdisciplinary education are gaining momentum, making computational modeling more accessible and effective for guiding difficult chemical synthesis.
- Google’s new AI video editor is now available for testing
- Trees and Air Quality: New Study Reveals Impact of Urban Greenery in LA
- Human footprints in New Mexico really may be surprisingly ancient, new dating shows
The Future of Computational Modeling in Chemistry
The future of computational modeling in chemistry looks promising, with numerous advancements on the horizon that could revolutionize the field. One of the most exciting trends is the integration of artificial intelligence (AI) and machine learning (ML) with traditional modeling techniques. These technologies have the potential to enhance predictive accuracy and streamline the design of complex chemical processes. By training algorithms on vast datasets, researchers can uncover patterns and predict outcomes that were previously unattainable with conventional methods.
Another significant development is the increasing computational power available through high-performance computing (HPC) and cloud-based platforms. These resources allow scientists to run more detailed and extensive simulations than ever before, providing deeper insights into molecular interactions and reaction mechanisms. The accessibility of these platforms also democratizes the field, enabling researchers from various institutions to contribute to and benefit from advanced computational tools.
Emerging applications of computational modeling are also expanding its impact. For instance, the development of green chemistry solutions, which focus on reducing environmental harm, can greatly benefit from precise modeling of sustainable processes and materials. Additionally, in pharmaceuticals, computational models are aiding in the rapid design and optimization of new drugs, significantly shortening development timelines and improving success rates.
Ongoing research and development remain crucial for further advancements. Continuous improvements in algorithms, software, and hardware are essential to keep pace with the growing complexity of chemical problems. Collaborative efforts across disciplines, including chemistry, computer science, and engineering, are vital to drive innovation and address the challenges of modern chemical synthesis.
The future of computational modeling in chemistry is undoubtedly bright, with the potential to transform not only how we understand chemical processes but also how we approach the synthesis of new compounds. As technological advancements continue to unfold, the field will likely witness unprecedented growth and innovation, paving the way for groundbreaking discoveries and applications.
Conclusion: Transforming Chemical Synthesis
Throughout this blog post, we have explored the pivotal role that computational modeling plays in the evolving landscape of chemical synthesis. By simulating complex reactions, scientists are able to predict outcomes and optimize processes that were once considered too challenging to undertake. This innovative approach significantly reduces trial and error, saving both time and resources in the laboratory.
Computational modeling has emerged as an indispensable tool, providing molecular-level insights that guide chemists through the intricate pathways of difficult chemical reactions. The ability to visualize and manipulate molecules in a virtual environment allows researchers to foresee potential obstacles and devise strategies to overcome them, thus streamlining the synthesis process.
Moreover, the integration of computational techniques with traditional experimental methods fosters a more comprehensive understanding of reaction mechanisms. This synergy not only enhances the precision of synthetic chemistry but also opens up new avenues for the discovery of novel compounds and materials with unprecedented properties.
As we look to the future, the continued advancement of computational modeling promises to further revolutionize the field of chemical synthesis. With ongoing improvements in computational power and algorithmic sophistication, the capability to model increasingly complex systems will expand. This will undoubtedly lead to more efficient synthetic routes, the development of innovative materials, and a deeper understanding of fundamental chemical principles.
Computational modeling is transforming chemical synthesis by making previously daunting reactions more accessible and manageable. Its impact is profound, setting the stage for groundbreaking discoveries and advancements in chemistry. The future of chemical synthesis is indeed bright, with computational modeling at the forefront of this scientific revolution.
FAQ: Computational Modeling Guides Difficult Chemical Synthesis
What is the challenge scientists are facing?
Traditionally synthesizing certain molecules, particularly those with specific ring structures, has been difficult and inefficient. This can slow down research and development in fields like pharmaceuticals.
How is computational modeling helping?
Scientists have developed computer models that can predict how different molecules will react under specific conditions. This allows them to virtually screen potential starting materials (reactants) to see if they have a high probability of forming the desired molecule in a good yield.
What specific type of molecule were the researchers focusing on?
This research focused on a class of molecules called azetidines, which have a four-membered ring containing nitrogen. These molecules have potential applications in drug development but have been challenging to synthesize compared to similar five-membered ring structures.
What was the benefit of using this approach?
By using computational modeling, researchers could quickly identify promising reactant pairs without needing to perform numerous lab experiments. This saves time, resources, and allows for a more targeted approach to synthesis.
Could this technique be applied to other types of chemical synthesis?
Yes, the researchers believe this approach has the potential to be broadly applicable to guide the synthesis of various complex molecules.
What are the next steps for this research?
The researchers will likely continue to refine their computational models and explore their application to a wider range of challenging syntheses. Additionally, they may collaborate with experimental chemists to validate their predictions in the lab and optimize reaction conditions.